
농경지 아산화질소 배출계수의 연간 변동 특성 분석
Abstract
We aimed at investigating the difference in N2O emission factors of chemical and organic fertilizers and identifying the main factors influencing annual fluctuations in N2O emission. We conducted two-year experiments in 2016 and 2017 in an agricultural field planted with sweet potato (Ipomoea batatas). Treatments included chemical NPK fertilizer (NPK) and chicken compost application at 10 ton ha-1, 20 ton ha-1, and 30 ton ha-1 rates (CK1, CK2 and CK3). Control was also employed with no addition. Results showed that N2O emission rates were significantly related with soil water status and soil available N contents. Significant correlation between % water filled pore space (WFPS) and N2O emission was observed only when the %WFPS was greater than 40% and during the initial stage of the experiment (<60 d). Comparison of the emission factors in 2016 and 2017 showed us that the emission factor was greater in 2016 when the %WFPS was maintained higher by 16.5% compared to that in 2017. In 2016, the emission factor of organic fertilizer was higher than that of chemical fertilizer, while in 2017, the pattern was reversed. Annual variability in N2O emission could also be originated from the available N contents remaining in soil after being taken up by plants. If we apply excessive N fertilizer, the soil would contain excess amount of N which was not uptaken by plants, leading to a huge increase in N2O emission. This case would overestimate emission factor, which was the case for the organic fertilizer in 2016. Over-fertilization should be avoided when we set up an experiment to determine N2O emission factor.
Keywords:
N2O Emission, Emission Factor, Organic Fertilizer, Chicken Compost, Over-Fertilization1. 서 론
주요 온실가스 중 하나인 아산화질소(N2O)는 인위적 배출원은 농경지에서 주로 배출되며, 전세계 총 배출량의 약 약 70%를 차지한다(Smith et al., 2008; Bouwman et al., 2010). 국가 온실가스 인벤토리는 농경지에서 배출되는 아산화질소를 포함하고 있으며, 이를 IPCC(2006)에서는 직접배출과 간접배출로 나누어 보고 있다. 직접배출은 토양에 투입된 질소 투입에 의해 토양으로부터 배출되는 아산화질소를 의미하고, 간접배출은 대기로 휘산된 암모니아나 질소산화물이 지표로 침적되거나, 토양으로부터 유출된 과잉 질소가 수계로 유입되어 일어나는 배출을 의미한다(IPCC, 2006). 이 중 직접배출에 의한 아산화질소는 배출계수(Emission Factor;l EF)를 사용하여 산정되는데, 그 동안 국내 배출계수는 주로 화학비료 사용에 대해 고추, 콩, 감자, 봄배추, 가을배추 등의 작물을 대상으로 개발되었다(Jeong et al., 2014; Kim et al., 2015).
하지만 화학비료 사용에 대한 문제점을 극복하고, 자원 순환적 토양 유기물을 관리하기 위하여 현 관행농법에서는 화학비료와 함께 퇴비를 처리하는 경우가 많다. 또한 IPCC(2006)의 Tier 1 방법에서는 화학비료에 대한 배출계수 이외에도 퇴비, 작물 부산물 및 토양 유기물에 대한 배출계수를 분리하여 포함시키고 있다. 한편, 화학비료에 기인한 아산화질소와 퇴비부터의 아산화질소는 그 배출기작에 영향을 주는 요인이 다를 수 있기 때문에 퇴비에 대한 배출계수는 분리해서 도출해야 한다(Hayakawa et al., 2009). 일반적으로 비료의 종류는 아산화질소 배출에 매우 큰 영향을 미치는데, 질산태 질소기반의 화학비료보다 암모늄태 질소기반의 화학비료가 아산화질소를 더 많이 배출하고, 동물 분변을 기반으로 한 퇴비 투입 시, 또는 화학비료와 퇴비를 함께 투입한 경우 배출량이 크다고 보고된다(Bouwman et al., 2002).
토양으로부터의 아산화질소 배출은 토양 미생물에 의해 이루어지는데, 질산화와 탈질이 주요 기작이다(Singh et al., 2010; Venterea et al., 2015). 미생물에 의한 아산화질소 배출은 토양의 무기태 질소량에만 영향을 받는 것이 아니라, 다른 환경적 요인, 특히 산소 조건에 큰 영향을 받는다(Robertson, 1989; Sánchez-García et al., 2014; Li et al., 2015, Lin et al., 2017). 이 때문에 아산화질소 배출은 시간적 공간적인 변이가 무척 큰데(Mosier, 1998), 특히 몽골이나 호주와 같은 반건조 지역에서는 연간 배출량의 변동폭은 평균치의 약 15배에 달한다고 보고되었다(Du et al., 2006). 이처럼 큰 연간 변동은 작물이 식재되지 않은 겨울철에 이상 기후에 따라 토양이 동결/해동을 반복하기 때문이라는 관찰도 있지만(Flessa et al., 1995), 화학 비료의 종류와 토지이용 형태, 그리고 기상환경의 변동을 그 큰 이유로 들고 있다(Scheer et al., 2008).
이처럼 아산화질소의 연간 변동이 크다는 점을 고려할 때, 국가별 신뢰성 있는 배출계수를 개발하기 위해서는 최소 3년 이상의 실험 반복과 연간 변동을 가져오는 주요한 인자를 도출해 내는 것이 필요하다. 하지만 아직까지 국내에서 퇴비 투입에 따른 아산화질소의 연간 배출 변동을 보고하고 분석한 사례는 없다. 이에 본 연구에서는 동일한 농경지에서 동일한 종류 및 양의 퇴비를 2년 연속 투입하였을 때에 연간 아산화질소 배출량의 변동을 파악하기 위해 야외 실험을 실시하였다. 연구의 목적은 1) 화학비료 투입에 따른 배출계수와 퇴비 투입에 따른 배출계수를 비교하고, 2) 연간 아산화질소 배출량의 변동을 정량화하며, 3) 배출량 변동에 영향을 주는 인자를 파악하는 것이다.
2. 재료 및 방법
2.1 야외 실험지 조성 및 처리구
본 실험은 충청남도 예산군 신암면 종경리에 위치한 충청남도 농업기술원에서 수행되었다. 실험은 2016년과 2017년 두 번에 걸쳐 5월에서 9월 사이 총 120일 재배기간 동안 진행되었는데, 처리구의 종류는 어떠한 처리도 하지 않은 무처리구(CON), N(urea)-P2O5-K2O 비료를 55-63-156 kg ha-1만큼 투입한 화학비료 처리구(NPK), 그리고 퇴비 처리구로 구성되었다. 퇴비로는 계분 퇴비를 사용하였는데, NPK 처리구와 동량의 화학비료를 처리한 이후 추가로 퇴비 10 ton ha-1를 투입한 CK1 처리구와 20 ton ha-1를 투입한 CK2 처리구, 30 ton ha-1를 투입한 CK3 처리구가 있다. 각 처리구의 면적은 10 m2(2.5 m × 4 m)로 한 처리당 3반복씩 수행하여 총 12 처리구를 조성하였고, 배치는 난괴법에 따랐다. 2016년 실험에서는 퇴비 처리구로 CK1과 CK3를 활용하였고, 2017년 실험에서는 CK1과 CK2를 활용하였다. 비료와 퇴비 투입은 2016년 5월 9일, 2017년은 4월 27일에 실시하였고, 그 이후에는 경운 로터리를 실시하고 두둑을 설치하였다. 이후 기체 포집용 챔버를 설치하고 밤호박 고구마[베니하루까 중만생종(Ipomoea batatas)]를 2016년 5월 11일, 2017년 5월 11일에 각각 심었으며, 추가적인 관개는 하지 않았다. 실험기간 동안 일 강수량, 일 평균 온도 등의 기상자료는 기상청 지역별상세관측자료(AWS, http://www.kma.go.kr/weather/observation/aws_table_ popup.jsp)를 참고하였으며, 관측 장소는 충청남도 예산군 신암면 종경리로 실험장소와 동일하다.
2.2 토양 및 퇴비 분석
기초 토양의 성상분석을 위하여 2016년 4월 20일과 2017년 4월 12일에 실시하였으며, 이후 토양분석은 토양기체를 채취하는 시점에 실시하였고, 0∼15 cm 깊이에서 코어 샘플러(직경 9.8 cm, Forest supplier, Inc., USA)를 이용하여 토양을 채취하였다. 채취한 샘플은 밀봉 후 실험실로 가져와서 수분함량을 측정하였고, 용적밀도를 계산하였다. 토성분석은 비중계법을 이용해 측정하였고, 미국농무부(USDA)의 기준에 따라 분류했다. pH는 1:5(w/v) 비율로 토양과 증류수를 혼합하고, 1시간 교반 후 유리전극(Orion 3star, Thermo, USA)을 이용하여 측정했다. EC는 Portable conductivity meters(Orion star A222, Thermo, USA)를 이용하여 측정했다. NH4+과 NO3- 농도는 salicylate microplate method(Sims et al., 1995)를 통해 측정했으며, 유기물 함량은 Ben-Dor and Banin(1989)의 Loss-On-Ignition method를 응용하여 측정했다.
퇴비는 전라북도 익산시에 위치한 신농비료에서 계분 퇴비를 구입하여 사용하였다. 동일한 농가에서 생산된 퇴비일지라도 연도별로 성상이 동일하지 않으므로 퇴비분석을 매년 수행하였다. 퇴비 내 총 탄소(TC)와 총 질소(TN)량을 원소분석기(Carlo Erba NS 1500 C/N analyzer, Carlo Erba, Milan, Italy)를 이용해 분석하였다.
2.3 아산화질소 측정 배출계수 계산
기체 샘플은 1주 또는 2주 간격으로 채취하였으며, 챔버법을 이용했다(Wang et al., 2011). 사용한 챔버는 하단부(지름 25 cm ⨉ 높이 38.5 cm)와 뚜껑부(지름 24 cm ⨉ 높이 24.5 m) 2개 부분으로 나뉘며, 하단부는 실험지를 조성할 때 각 처리구 별로 한 개씩 5 cm 깊이로 설치해 두었다. 기체 채취일에는 뚜껑부로 하단부를 덮어 밀폐시킨 후 40분 간 headspace에 포집한 기체를 10 mL 주사기를 이용해 채취하였다. 채취한 기체 시료는 바로 실험실로 운반하여 Agilent Technology 7890A Gas Chromatograph(Agilent Technology, Inc., USA)를 이용하여 분석하였으며, 아산화질소 분석에는 electron capture detector (ECD)를 사용했다. Gas flux는 Troy et al.(2013)의 식을 이용하여 산출하였다.
(eq.1) |
는 초기 아산화질소 농도와 40분 후 채취한 시료의 아산화질소 농도 차이며(ppb day-1), V는 챔버의 부피(m3), A는 챔버의 단면적(m2)이다. ρ는 아산화질소의 가스밀도(mg m-3)이며, T는 챔버의 온도(℃)이다. 최종 flux의 단위는 mg m-2 day-1이다.
NPK 처리구에서의 아산화질소 배출 계수는 eq.2를 사용해 계산하였고, 퇴비의 배출계수 계산을 위해서는 CK1, CK2, CK3 처리구의 연간 누적 아산화질소 배출량에서 NPK 처리구의 연간 누적 아산화질소 배출량을 각각 뺀 값을 y축으로 하고, 처리구별 투입된 퇴비 기인 질소량을 x 축으로 하여 연별 3지점으로 회귀 직선을 그려 그 기울기를 배출 계수로 하였다(Charles et al., 2017).
(eq.2) |
는 NPK 처리구와 대조구의 연간 누적 아산화질소 배출량 차이를 의미하고, 는 NPK 처처리구의 질소 투입량을 의미하며, 은 0이다.
2.4 통계 분석
통계분석은 SAS 9.4(SAS Institute, USA, 2013)의 일반선형모델(general linear model; GLM)을 이용하여 처리구별 5% 유의수준에서 분산분석을 수행하였다. 분석은 처리구간 아산화질소 배출량 차이, 비료와 계분 처리에 따른 연별 아산화질소 배출량 차이에 대해 실시하였다. 회귀식과 결정계수 R2는 SigmaPlot 12.5(Systat Software Inc. UK)를 이용해 도출했으며, WFPS와 아산화질소 배출속도, 질소 투입량과 누적 아산화질소 배출량에 대해 수행하였다.
3. 결과 및 토의
3.1 기초 토양성상 및 투입물질 분석
대상 토양의 pH는 6.9로 중성이고, 유기물 함량은 1.6%이며, 토성은 미사질 양토이다(Table 1). 투입 물질인 계분을 이용한 퇴비의 성상은 Table 2와 같다. 2016년 및 2017년 동일한 농가에서 구입한 계분임에도 불구하고 성상의 차이가 크게 발생하였고, C/N비는 2016년이 2017년에 비해 약 2배 높은 것을 알 수 있다.
3.2 아산화질소 배출
시간에 따른 기온, 강수량 그리고 % WFPS 및 아산화질소 배출속도의 변화는 2016년과 2017년 각각 Fig. 2과 Fig. 3에 나타내었다. 일반적으로 아산화질소의 배출은 토양의 % WFPS와 유사한 패턴을 보였는데, 이는 Khalil and Baggs(2005), Skiba and Smith(2000)의 연구에서도 관찰되었다. 하지만 토양 % WFPS와 아산화질소 배출량 간 유의한 상관 관계는 오직 % WFPS가 40% 이상이면서 실험 시작 후 79일 이전에만 존재하였는데(Fig. 5), 이 때의 상관계수는 0.67(p<0.001)로 매우 높았다. 이는 아산화질소 배출에 수분 이외의 요인이 함께 작용하고 있음을 의미한다. 그 중 하나는 토양 내의 무기태 질소 함량이다. 무기태 질소 함량은 비료 투입 후 약 79일까지 높게 유지되다가 그 이후 현격히 줄어드는 경향을 보였다(Fig. 4). Fig. 2에서 2016년에는 화학 비료 시비로 인해 토양 내 무기태 질소 함량이 높게 유지될 때에 비가 내려 토양의 수분함량이 높았으므로, 실험 실시 후 79일 이내에 아산화질소 배출량은 전체 누적 배출량의 약 76%를 차지하였다. 반면, 2017년에는 시비 직후에 비가 오지 않아 토양 % WFPS가 30% 이하로 낮게 유지되었으므로 2016년도처럼 아산화질소 배출속도의 급격한 증가가 관찰되지 않았다. 하지만 재배기간의 후반기에는 토양의 % WFPS가 높다 하더라도 아산화질소 배출이 그다지 높지 않거나 전혀 증가하지 않았는데, 이는 투입한 화학 비료 성분이 초반부에 이미 휘산되었거나, 질산화를 통해 질산태 질소로 전환되었기 때문으로 사료된다(Rawluk et al., 2001; Di and Cameron, 2004).
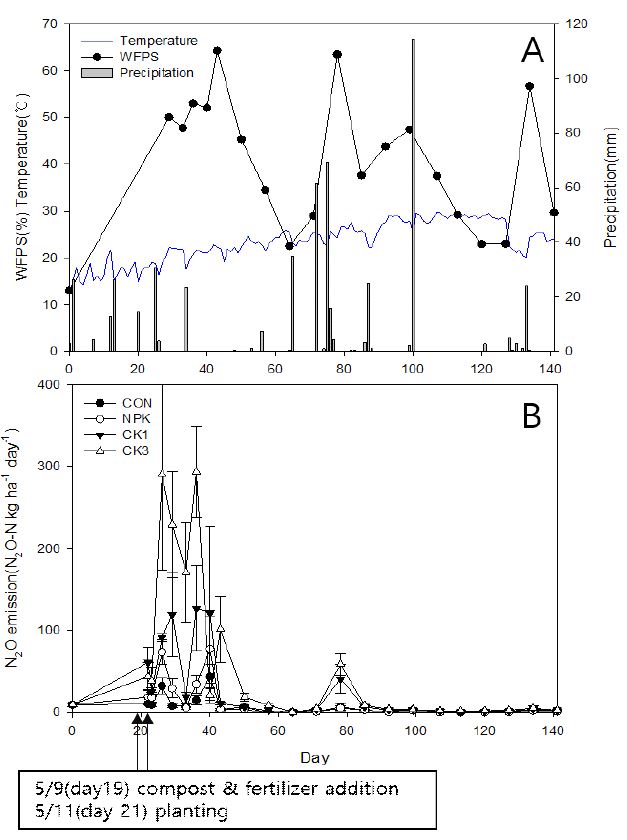
Temporal change in % water filled pore space (% WFPS), temperature, precipitation, and N2O emission rates from the treatments and control in 2016. Day 0 on x axis indicates the day of soil sampling for basic characterization.
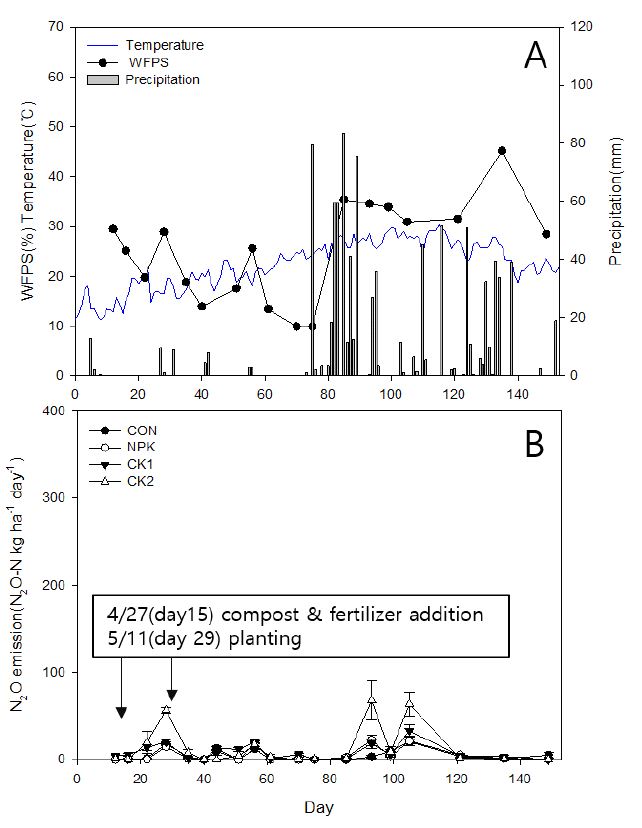
Temporal change in % water filled pore space (% WFPS), temperature, precipitation, and N2O emission rates from the treatments and control in 2017. Day 0 on x axis indicates the day of soil sampling for basic characterization.
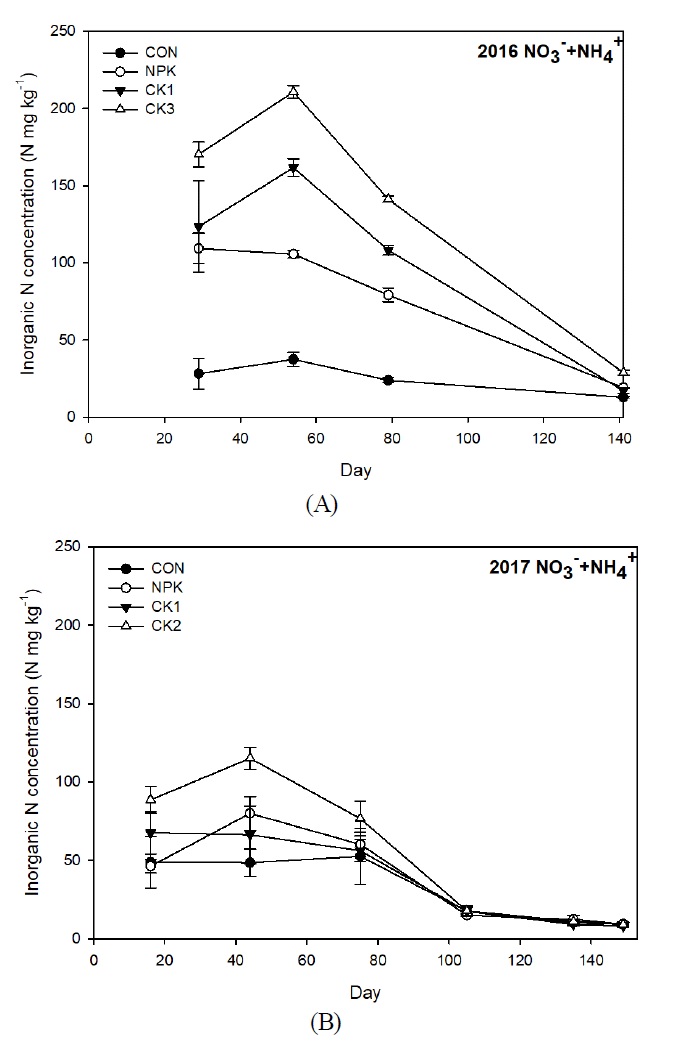
Temporal change in inorganic nitrogen concentration(NH4-N+ NO3-N) in the treatments and control in A 2016 and B 2017.
3.3 누적 아산화질소 배출량 및 배출계수 연간 비교
2016년과 2017년의 누적 아산화질소 배출량 비교는 Fig. 6과 같다. CON, NPK, CK1의 경우는 2016년과 2017년의 누적 아산화질소 배출량의 유의한 차이는 없다. 이는 2017년 퇴비의 C/N비가 2016년의 거의 1/2배에 불과하다는 사실을 고려하면 예상 밖의 결과이다. 이는 아산화질소 배출에 영향을 주는 인자는 여러 가지이지만, 토양 내에 질소 함량이 충분히 높다 하더라도 토양 수분함량이 이를 촉발하는 직접적인 요인이라는 점을 시사한다. 즉, 토양의 질소 함량과 수분조건이 동시에 충족되어야 아산화질소의 배출이 일어날 수 있다.
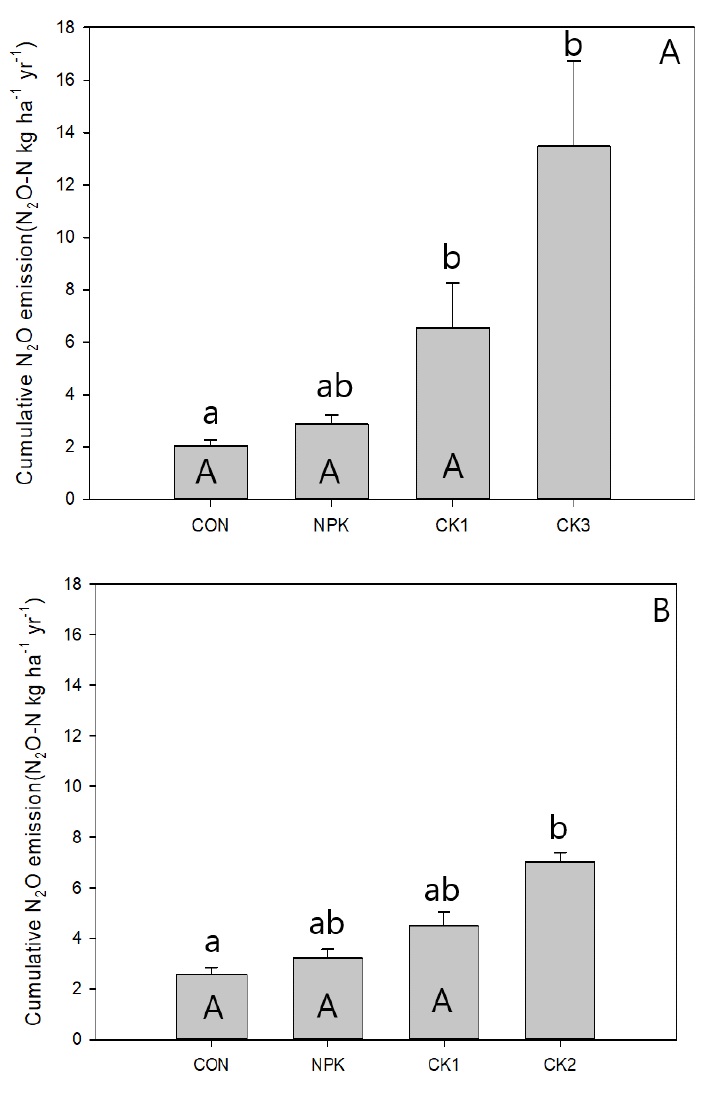
Cumulative annual N2O emissions from the treaments and control in A) 2016 and B) 2017. Bars with different lower case letters indicate significant differences among the treatments within the same year. Bars with different upper case letters indicate significant difference between year 2016 and 2017 within the same treatment.
연도별 배출계수는 2016년에 비하여 2017년이 NPK 및 CK 처리구에서 각각 25% 및 59% 만큼 높은 것을 알 수 있다(Table 3). 또한 2016년에는 화학비료에 의한 배출계수보다 퇴비에 대한 배출계수가 약 27% 더 높았으나, 2017년에는 퇴비에 대한 배출계수가 오히려 30% 감소하였다. 2016년과 같은 경향은 Hayakawa et al.(2009)의 연구에서도 발견되는데, 이는 퇴비에서 추가적으로 가용한 질소가 유입되었기 때문이다. 하지만 2017년처럼 퇴비의 배출계수가 화학비료보다 적은 경우도 있는데, Cai et al.(2013)에서는 그 이유를 화학비료에 비해 퇴비의 무기태 질소가 더 적기 때문이라고 설명하고 있다. 연도별 배출계수의 차이는 앞서 기술하였듯이 기상 인자와 토양 내 무기태 질소량으로 설명할 수 있다. NPK 처리구에서 질소 함량은 2년 간 동일하게 투입하였으므로 배출계수의 차이를 대부분 기상인자, 특히 토양수분함량으로 설명할 수 있다. 하지만 CK 처리구에서 배출계수의 연도별 차이는 기상인자 이외에 다른 요인이 있을 텐데, 이는 토양 내 잔존 무기질소 함량이다. CK 처리구에 있어서 2016년과 2017년 사이의 가장 큰 차이는 2016년 CK3 처리구 대신 2017년 CK2 처리구로 바꾸었다는 점이다. 만약, 여기서 식물에 의한 질소 흡수량이 투입된 질소량에 비례한다면, 토양 내 잔존 질소량도 투입량에 선형으로 증가할 것이고 이에 따른 아산화질소 배출량도 비례할 것이다. 그러나 식물의 질소 흡수량에는 상한선이 있고, 요구량 이상의 질소가 토양에 존재한다고 해도 이를 모두 흡수하지는 않는다. 과량의 질소는 토양에 잔존하게 되고 이 때문에 아산화질소의 배출량이 선형이 아닌 지수 함수적으로 증가하여 배출계수가 과대평가되었을 수가 있다. 이와 같은 관계는 Fig. 7에 잘 나타나 있다. 2016년과 2017년 자료를 통합하여 회귀식을 그려보면 선형관계에 따른 결정식이 0.7373인 반면, 지수함수 관계에 따른 결정식은 0.8918로 약 21% 개선되는 것을 알 수 있다. 이 결과가 의미하는 바는 배출계수를 도출하기 위한 실험을 계획할 때에 작물의 질소 요구량을 고려하여 너무 과도한 잉여 질소가 토양에 축적되지 않도록 하는 고려가 필요하다는 점이다.
4. 결 론
본 연구는 국내에서 퇴비에 대한 아산화질소 배출계수를 도출할 때 고려해야 할 주요 인자에 대한 시사점을 도출하였다. IPCC의 가이드라인에서도 명시되어 있듯이 아산화질소의 배출은 연간 변동이 크기 때문에 최소 3년의 자료를 평균하여 사용하도록 되어 있다. 연간 변동의 원인은 주로 기상인자와 토양 내 잔존 무기태 질소량인데, 기상인자의 경우는 시비 직후의 토양 수분 함량이 아산화질소 배출의 가장 주요한 인자로 파악되었다. 토양 내 잔존 무기태 질소에서 고려할 점은 식물의 질소 요구량이다. 질소 요구량이 낮은 작물의 아산화질소 배출계수를 도출하려 할 때에는 이를 고려하여 과도한 비료 투입을 자제해야 한다. 과도한 비료 투입은 질소량과 아산화질소 발생량 사이의 선형 관계식을 무너뜨리고 지수 함수적인 아산화질소의 증가를 가져오므로 배출계수가 과대평가될 우려가 있다. 특히 퇴비의 경우는 연간 비료 성상의 변동이 크고, 이에 따른 무기태 질소량에도 차이가 생길 수 있기 때문에 화학비료에 따른 배출계수를 도출할 때보다 더 세심한 관리가 필요하다.
Acknowledgments
본 연구는 농촌진흥청 국립농업과학원 농업과학기술 연구개발사업(과제번호: PJ0118552017)의 지원에 의해 수행되었습니다.
References
- Ben-Dor, E., Banin, A., (1989), of organic matter content in arid-zone soils using a simple “loss-on-ignition” method, Commun Soil Sci Plant Anal, 20, p1675-1695.
-
Bouwman, AF., Boumans, LJM., Batjes, NH., (2002), Modeling global annual N2O and NO emissions from fertilized fields, Globa lBiogeochem. Cycles, 16, p28-1-28-9.
[https://doi.org/10.1029/2001gb001812]
- Bouwman, AF., Stehfest, E., Kessel van, C., (2010), Nitrous oxide emissions from the nitrogen cycle in arable agriculture: Estimation and mitigation, in: Nitrous Oxide and Climate Change, p85-106.
-
Charles, A., Rochette, P., Whalen, JK., Angers, DA., Chantigny, MH., Bertrand, N., (2017), Global nitrous oxide emission factors from agricultural soils after addition of organic amendments: A meta-analysis, Agric Ecosyst Environ, 236, p88-98.
[https://doi.org/10.1016/j.agee.2016.11.021]
-
Cai, Y., Ding, W., Luo, J., (2013), Nitrous oxide emissions from Chinese maize-wheat rotation systems: A 3-year field measurement, Atmos Environ, 65, p112-122.
[https://doi.org/10.1016/j.atmosenv.2012.10.038]
-
Di, HJ., Cameron, KC., (2004), Effects of temperature and application rate of a nitrification inhibitor, dicyandiamide (DCD), on nitrification rate and microbial biomass in a grazed pasture soil, Aust J Soil Res, 42, p927-932.
[https://doi.org/10.1071/sr04050]
- Du, R., Lu, D., Wang, G., (2006), Diurnal, seasonal, and inter-annual variations of N2O fluxes from native semi-arid grassland soils of inner Mongo, Soil Biol Biochem, 38, p3474-3482.
- Flessa, H., Dörsch, P., Beese, F., (1995), Seasonal variation of N2O and CH4 fluxes in differently managed arable soils in southern Germany farmyard manure (12 t ha-l). The intensively managed field was planted with spring wheat and fertilized with a total of 190 kg N ha-1 given as calcium ammo, J Geophys Res, 100, p115-124.
-
Hayakawa, A., Akiyama, H., Sudo, S., Yagi, K., (2009), N2O and NO emissions from an Andisol field as influenced by pelleted poultry manure, Soil Biol Biochem, 41, p521-529.
[https://doi.org/10.1016/j.soilbio.2008.12.011]
- Intergovernmental Panel on Climate Change (IPCC), Eggleston, HS., Buendia, L., Miwa, K., Ngara, T., Tanabe, K., (2006), Agriculture, forestry and other land use. IPCC guidelines for national greenhouse gas inventories, Institute for Global Environmental Strategies, Hayama, Japan, 2, p48-56.
-
Jeong, HC., Lee, JS., Choi, EJ., Kim, GY., Seo, SU., So, KH., (2014), A comparison of the changes of greenhouse gas emissions to the develop country-specific emission factors and scaling factors in agricultural sector, J Clim Chang Res, 5, p349-357.
[https://doi.org/10.15531/ksccr.2014.5.4.349]
-
Khalil, MI., Baggs, EM., (2005), CH4 oxidation and N2O emissions at varied soil water-filled pore spaces and headspace CH4 concentrations, Soil Biol Biochem, 37, p1785-1794.
[https://doi.org/10.1016/j.soilbio.2005.02.012]
- Kim, GY., Park, WK., Jeong, HC., Lee, SI., Choi, EJ., Kim, PJ., Seo, YH., Na, US., (2015), A revised estimate of N2O emission factor for spring Chinese cabbage fields in Korea, Korean Journal of Agricultural and Forest Meteorology, 17, p326-332.
- Li, B., Fan, CH., Xiong, ZQ., Li, Q., Zhang, M., (2015), The combined effects of nitrification inhibitor and biochar incorporation on yield-scaled N2O emissions from an intensively managed vegetable field in southeastern China, Biogeosciences, 12, p2003-2017.
- Lin, Y., Ding, W., Liu, D., He, T., Yoo, G., Yuan, J., Chen, Z., Fan, J., (2017), Wheat straw-derived biochar amendment stimulated N2O emissions from rice paddy soils by regulating the amoA genes of ammonia-oxidizing bacteria, Soil Biol Biochem, 113, p89-98.
- Mosier, AR., (1998), Soil processes and global change, Biol Fertil Soils, 27, p221-229.
-
Rawluk, CDL., Grant, C., Racz, GJ., (2001), Ammonia volatilization from soils fertilized with urea and varying rates of urease inhibitor NBPT, Can J Soil Sci, 81, p239-246.
[https://doi.org/10.4141/s00-052]
- Robertson, GP., (1989), Nitrification and denitrification in humid tropical ecosystems: Potential controls on nitrogen retention, Miner Nutr Trop For savanna Ecosyst, p55-69.
- Sánchez-García, M., Roig, A., Sánchez-Monedero, MA., Cayuela, ML., (2014), Biochar increases soil N2O emissions produced by nitrification-mediated pathways, Front Environ Sci, 2, p1-10.
- Scheer, C., Wassmann, R., Kienzler, K., Ibragimov, N., Eschanov, R., (2008), oxide emissions from fertilized, irrigated cotton (Gossypium hirsutum L.) in the Aral Sea Basin, Uzbekistan: Influence of nitrogen applications and irrigation practices, Soil Biol Biochem, 40, p290-301.
-
Sims, GK., Ellsworth, TR., Mulvaney, RL., (1995), Microscale determination of inorganic nitrogen in water and soil extracts, Commun Soil Sci Plant Anal, 26, p303-316.
[https://doi.org/10.1080/00103629509369298]
-
Singh, BP., Hatton, BJ., Singh, B., Cowie, AL., Kathuria, A., (2010), Influence of biochars on nitrous oxide emission and nitrogen leaching from two contrasting soils, J Environ Qual, 39, p1224-1235.
[https://doi.org/10.2134/jeq2009.0138]
-
Skiba, U., Smith, KA., (2000), The control of nitrous oxide emissions from agricultural and natural soils, Chemosph Glob Chang Sci, 2, p379-386.
[https://doi.org/10.1016/s1465-9972(00)00016-7]
- Smith, P., Martino, D., Cai, Z., Gwary, D., Janzen, H., Kumar, P., McCarl, B., Ogle, S., O’Mara, F., Rice, C., Scholes, B., Sirotenko, O., Howden, M., McAllister, T., Pan, G., Romanenkov, V., Schneider, U., Towprayoon, S., Wattenbach, M., Smith, JU., (2008), Greenhouse gas mitigation in agriculture, Philos Trans R Soc Lond B Biol Sci, 363, p789-813.
-
Troy, SM., Lawlor, PG., O’ Flynn, CJ., Healy, MG., (2013), Impact of biochar addition to soil on greenhouse gas emissions following pig manure application, Soil Biol Biochem, 60, p173-181.
[https://doi.org/10.1016/j.soilbio.2013.01.019]
-
Venterea, RT., Clough, TJ., Coulter, JA., Breuillin-Sessoms, F., (2015), Ammonium sorption and ammonia inhibition of nitrite-oxidizing bacteria explain contrasting soil N2O production, Sci Rep, 5, p12153.
[https://doi.org/10.1038/srep12153]
-
Wang, J., Zhang, M., Xiong, Z., Liu, P., Pan, G., (2011), Effects of biochar addition on N2O and CO2 emissions from two paddy soils, Biol Fertil Soils, 47, p887-896.
[https://doi.org/10.1007/s00374-011-0595-8]